First Experimental Proof of Theorized Nuclear Fusion Processes in Massive Stars
The international Borexino Collaboration has announced the first observation of neutrinos from the CNO process in the Sun, which experimentally confirms the second mechanism for energy generation in stars. Earlier, only neutrinos from the proton–proton cycle were observed. This discovery is highly important for astrophysics since in stars more massive than the Sun energy is mainly produced through the CNO cycle. The results of the investigation are published in Nature.
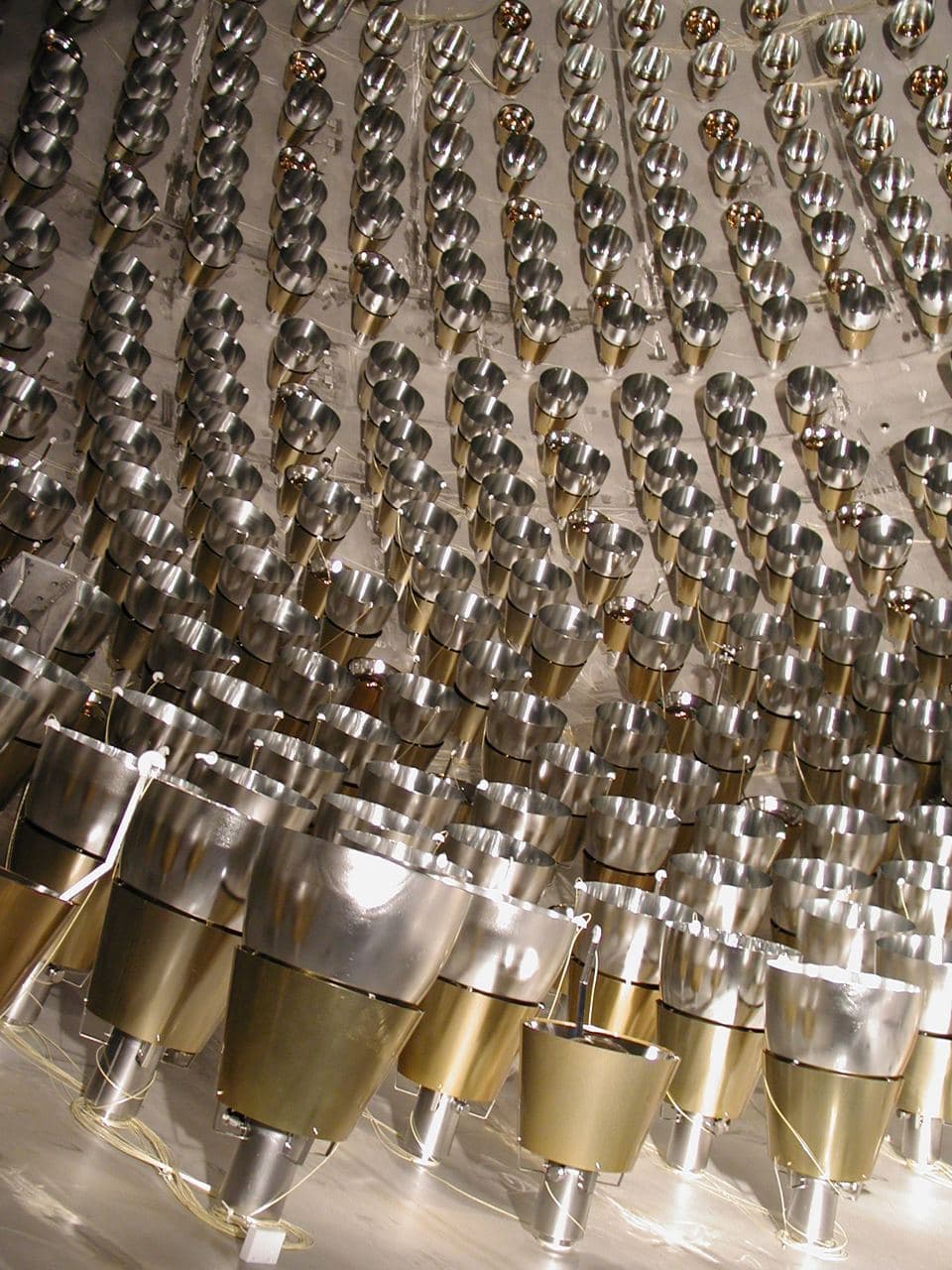
The international Borexino Collaboration has announced the first observation of neutrinos from the CNO process in the Sun, which experimentally confirms the second mechanism for energy generation in stars. Earlier, only neutrinos from the proton–proton cycle were observed. This discovery is highly important for astrophysics since in stars more massive than the Sun energy is mainly produced through the CNO cycle. The results of the investigation are published in Nature.
Text illustration by Alina Vishneva
The core of the Sun is a colossal thermonuclear reactor, where protons fuse into helium at a temperature of about 15 million degrees. Helium is produced via two multistage processes, the proton–proton (pp) chain and the CNO cycle. Some of the nuclear reactions are accompanied by emission of neutrinos. Because of their extremely low interaction probability with matter, neutrinos easily move through the Sun retaining information both on nuclear processes within the Sun and on their occurrence conditions. Though the solar neutrino flux is as immense as billions particles per square centimeter per second, detection of elusive neutrinos is a much complicated experimental problem.
CNO cycle in stars
In relatively light stars, including the Sun, the pp chain is the dominant mechanism in terms of the amount of generated energy. In heavier stars with a mass half again as large as the Sun’s mass, the CNO cycle dominates in generation of energy. Carbon, nitrogen, and oxygen catalyze reactions of the CNO cycle while ultimately remaining unchanged. Neutrinos emitted in the nuclear reactions serve as their characteristic tags. About 90% of neutrinos are emitted in the fusion of hydrogen nuclei. The second highest-intensity neutrino flux accounts for about 10% and results from electron capture of beryllium-7 in one of the later-stage pp chain reactions. Other neutrino fluxes are less intense.
“The Borexino detector was initially intended for detecting the beryllium neutrino flux, but its phenomenal characteristics made it also possible to detect neutrinos from other, less intense sources in the pp chain,” says Oleg Smirnov, Candidate of Sciences (Phys. Math.), Senior Researcher of DLNP, head of the Dubna team in the Borexino Collaboration. “Nevertheless, the question of whether it will be possible to detect neutrinos from the CNO cycle has remained an open question until recently. In addition to lowness of the flux itself, observation of CNO neutrinos is also hampered by the spectral component of the natural background indistinguishable from their spectrum.”
For the Sun, the amount of energy generated via the CNO cycle is relatively small, while for a great number of more massive stars in the universe and for their fast evolution the CNO cycle is the main mechanism for fusion of hydrogen into helium. Energy generation via the CNO cycle is well justified theoretically, but its occurrence in the Sun has not been experimentally proved yet.
Fig. 1. Photosensors (PMTs) of the Borexino detector on the inner surface of the metallic sphere. In front of each PMT there is a cone-shaped light concentrator. The open part of the concentrator is 50 cm in diameter. The yellow truncated cylinders protect the PMTs against the magnetic field of the Earth (Copyright: Borexino collaboration).
First experimental proof
The property to ignore matter allows neutrinos to keep information on internal processes in the Sun but also makes them unobservable for ordinary particle detectors. Therefore, specifically designed detectors with a very large mass and thorough monitoring of various processes imitating neutrino interactions are used to detect neutrinos. In the Borexino detector, the active medium is 100 t of liquid scintillator. Rarely, a neutrino interacts with an electron, transferring part of its energy to the latter. The process resembles collision of billiard balls. Having gained an initial velocity, the electron gradually loses the received energy in the interaction with molecules of the medium. Part of the transferred energy is emitted in the form of photons. Thus, the neutrino–electron interaction results in a flash of light, and a few thousand photons move in various directions away from the interaction point. Detection of these photons by special highly sensitive devices—photomultiplier tubes (PMTs)—allows estimating the energy transferred to the electron and determining the point of interaction in the detector.
“Despite a great number of solar neutrinos passing through the detector (over a sextillion a day), only some fifty of them leave a noticeable “footprint” in the detector. Scientists involved in the analysis of the data isolated a signal that could only be attributed to the CNO neutrino. Thus, it was proved that nuclear reactions of the CNO cycle occur in the Sun. The total CNO neutrino flux makes up about 1% of the total solar neutrino flux,” explains Oleg Smirnov.
Fig. 2. Interior of the Borexino detector with mounted PMTs (Copyright: Borexino collaboration).
Thousands of light detectors 1400 m below the Earth’s surface
The Borexino detector has been taking data almost continuously since May 2007. It is located in the world’s largest underground laboratory, the Gran Sasso National Laboratory (Central Italy). At the center of the detector there is a transparent vessel of very thin nylon stretched with a set of nylon strings. It contains about 300 t of special ultrapure fluid that emits light when traversed by charged particles. Weak light flashes are detected by 2000 large sensors (20-cm PMTs) mounted on the metallic sphere around the fiducial volume. The permissible radioactive contamination threshold of the liquid scintillator is billions of times lower than the ordinary radioactivity level of natural materials, e.g., water. Therefore, all fluids used in the experiment were deeply purified of natural radioactive contaminants. Radioactivity of detector materials was strictly controlled during the construction of the detector. The objective of searching for CNO neutrinos required complete thermal insulation of the detector against ambient influences to avoid formation of heat flows transferring residual radioactivity from the inner walls of the detector to its volume.
The rock overburden above the laboratory equivalent to 3800 m of water considerably suppresses the cosmic-ray flux. Nevertheless, cosmic radiation partially penetrates the rock, giving rise to undesirable backgrounds that hamper observation of neutrinos. Physicists developed special data analysis techniques to separate the neutrino and background signals.
The proof that CNO reactions occur in the Sun is an important scientific achievement, a step on the way to comprehending the chemical composition of the Sun. Since the neutrino flux produced in the CNO cycle is directly related to the concentration of the elements C, N, and O participating in the reactions, measurement of these neutrino fluxes is directly related to the chemical composition of the Sun.
The Borexino experiment has confirmed once again its leading position in astrophysical researches, revealing secrets of life-sustaining mechanisms for stars, including the Sun.
Fig. 3. Schematic view of the CNO cycle (from Wikipedia).
Borexino Collaboration
The Borexino Collaboration involves a total of about 100 researchers from Italy, Germany, the United States, Russia, France, and Poland. The DLNP (JINR) team, one of the earliest participants, has been in the project since the beginning of its implementation in 1991. Apart from the JINR scientists, participants in the Borexino project are our colleagues from other Russian institutes: the National Research Center “Kurchatov Institute” (Moscow), the Petersburg Nuclear Physics Institute of the NRC “Kurchatov Institute” (St. Petersburg), and the Skobeltsyn Institute of Nuclear Physics of Moscow State University (Moscow).
The Borexino detector has been taking data since 2007 and has been a source of important findings in solar and terrestrial neutrino physics.
Borexino team from Dubna
The Borexino team of the Joint Institute for Nuclear Research was set up in 1991 and has participated in the experiment since the very beginning. The team has made a considerable contribution to the investigation of solar neutrino fluxes, search for rare processes, and search for geoneutrinos.
Fig. 4. Exterior of the Borexino detector with thermal insulation installed (Copyright: Borexino collaboration).
Further information
Original publication:
https://www.nature.com/articles/s41586-020-2934-0
DOI 10.1038/s41586-020-2934-0
“Experimental evidence of neutrinos produced in the CNO fusion cycle in the Sun”
The Borexino Collaboration: M. Agostini, K. Altenmuller, S. Appel, V. Atroshchenko, Z. Bagdasarian, D. Basilico, G. Bellini, J. Benziger, R. Biondig, D. Bravo, B. Caccianiga, F. Calaprice, A. Caminata, P. Cavalcante, A. Chepurnov, D. D’Angelo, S. Davini, A. Derbin, A. Di Giacinto, V. Di Marcello, X.F. Ding, A. Di Ludovico, L. Di Noto, I. Drachnev, A. Formozov, D. Franco, C. Galbiati, C. Ghiano, M. Giammarchi, A. Goretti, A.S. Gottel, M. Gromov, D. Guffanti, Aldo Ianni, Andrea Ianni, A. Jany, D. Jeschke, V. Kobychev, G. Korga, S. Kumaran, M. Laubenstein, E. Litvinovich, P. Lombardi, I. Lomskaya, L. Ludhova, G. Lukyanchenko, L. Lukyanchenko, I. Machulin, J. Martyn, E. Meroni, M. Meyer, L. Miramonti, M. Misiaszek, V. Muratova, B. Neumair, M. Nieslony, R. Nugmanov, L. Oberauer, V. Orekhov, F. Ortica, M. Pallavicini, L. Papp, L. Pelicci, O. Penek, L. Pietrofaccia, N. Pilipenko, A. Pocar, G. Raikov, M.T. Ranalli, G. Ranucci, A. Razeto, A. Re, M. Redchuk, A. Romani, N. Rossi, S. Schonert, D. Semenov, G. Settanta, M. Skorokhvatov, A. Singhal, O. Smirnov, A. Sotnikov, Y. Suvorov, R. Tartaglia, G. Testera, J. Thurn, E. Unzhakov, F.L. Villante, A. Vishneva, R.B. Vogelaar, F. von Feilitzsch, M. Wojcik, M. Wurm, S. Zavatarelli, K. Zuber, G. Zuzel
Borexino experiment:
Gran Sasso National Laboratory: